Charge injection in MOSFETs occurs through mechanisms such as Hot electron injection and Fowler–Nordheim tunneling, each with distinct behaviors and implications for n-channel and p-channel devices.
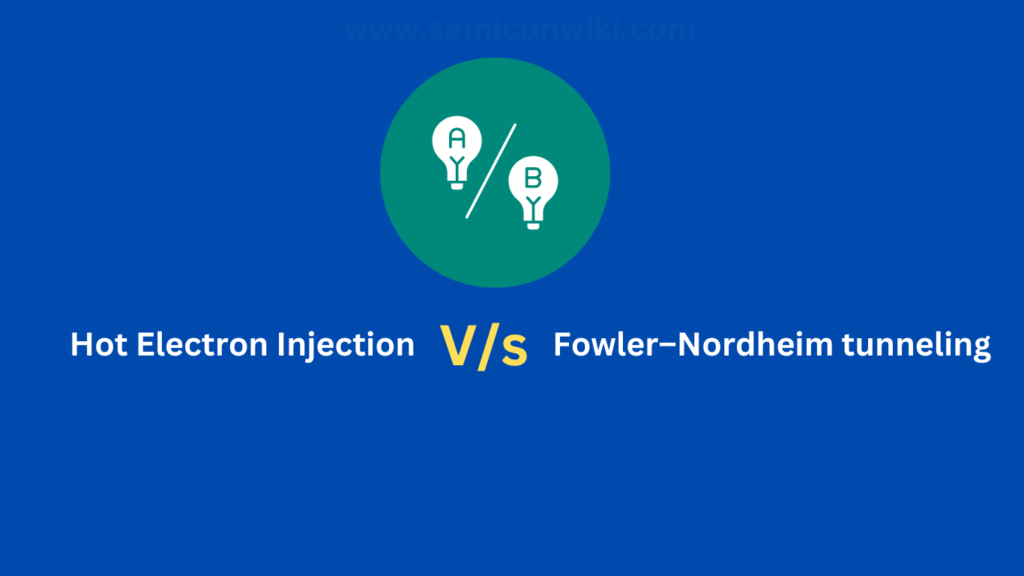
Hot Electron Injection
This process involves high-energy electrons overcoming potential barriers to enter the gate oxide. For n-channel MOSFETs, hot electron injection is primarily driven by channel electrons gaining energy under a high electric field. However, I observed that this method is inefficient in n-channel devices because the gate voltage required to maximize the electric field in the channel does not align well with the voltage favorable for gate insulator injection. You may notice that the maximum gate current in such cases is extremely low, as reflected by a maximum Ig/Ids ratio
On the other hand, we see a more efficient injection process in p-channel MOSFETs, where secondary hot electrons generated through avalanche multiplication facilitate electron injection into the gate insulator. The gate voltage that optimizes avalanche electron generation also supports injection into the gate, resulting in a higher gate current and a more favorable Ig/Ids ratio.
Avalanche Injection and Its Role
When a p-channel MOSFET operates with its source floating, the device acts as a gated diode, allowing injection without significant channel current. This contrasts with n-channel devices, where both hot electron and hot hole injections occur under different gate voltage conditions. I found it intriguing that at lower gate voltages, n-channel MOSFETs exhibit hot hole injection, while at higher voltages, hot electron injection becomes dominant. This interplay significantly impacts device performance and reliability.
Fowler–Nordheim Tunneling
For nonvolatile memory applications, Fowler–Nordheim tunneling provides an efficient alternative to hot electron injection. In this mechanism, electrons tunnel through the triangular energy barrier in the oxide layer. Since no unintended channel current flows during this process, the power dissipation is significantly lower. We often use oxide thickness around 10 nm and an electric field of 8–10 MV/cm to achieve a programming time between 1 μs and 1 ms. However, too thin an oxide risks direct tunneling and charge leakage, while too thick an oxide requires impractically high voltages.
Hot carrier effects can lead to unintended consequences, such as shifts in the threshold voltage (VtV_tVt) in neighboring cells of nonvolatile memories. For example, in n-channel memory devices, secondary hot hole injection can disturb unselected cells, potentially erasing stored data or causing leakage. You must design with care to avoid such issues by keeping wordline voltages well below the threshold for unselected devices.
In summary, while both hot carrier injection and Fowler–Nordheim tunneling enable charge injection, their efficiency, power requirements, and effects on device longevity vary significantly between n-channel and p-channel MOSFETs.